Table of Contents
What is Enzyme Inhibition?
- Enzyme inhibition is a fundamental biological process that involves the reduction or cessation of enzyme activity due to the presence of specific molecules known as enzyme inhibitors. These inhibitors play a pivotal role in regulating various metabolic pathways, ensuring cellular balance, and even serving as potential therapeutic agents. Therefore, understanding the intricacies of enzyme inhibition is crucial for both biological research and medical applications.
- Enzymes are specialized proteins that catalyze specific chemical reactions, transforming substrate molecules into products. They achieve this by binding the substrate to their active site, a unique region on the enzyme designed to facilitate the reaction. However, when an enzyme inhibitor is present, it can bind to the enzyme, preventing the substrate from accessing the active site or altering the enzyme’s structure, thereby inhibiting its catalytic function.
- There are two primary types of enzyme inhibitors: reversible and irreversible. Reversible inhibitors bind to the enzyme non-covalently, meaning they can detach, allowing the enzyme to regain its activity. On the other hand, irreversible inhibitors form a permanent bond with the enzyme, rendering it inactive unless the bond is broken.
- Besides, enzyme inhibitors can be classified based on their mode of action. Some inhibitors bind directly to the enzyme’s active site, competing with the substrate for access. Others bind to a different site on the enzyme, causing a conformational change that affects the enzyme’s ability to bind the substrate or catalyze the reaction.
- One of the key functions of enzyme inhibitors in cells is to regulate enzyme activity. For instance, in metabolic pathways, enzymes may be inhibited by molecules produced downstream in the pathway. This negative feedback mechanism ensures that cells do not produce excess molecules, maintaining cellular homeostasis. Additionally, enzyme inhibitors can protect cells from potential damage by controlling enzymes that might otherwise harm the cell if left unchecked.
- Furthermore, many natural toxins produced by plants and animals act as enzyme inhibitors, targeting specific enzymes in predators or prey. These inhibitors can disrupt vital physiological processes, leading to paralysis, respiratory failure, or even death.
- In the realm of medicine, enzyme inhibitors have found significant applications, especially as therapeutic agents. Many drugs are designed to inhibit specific enzymes, either to treat diseases caused by aberrant human enzymes or to target enzymes crucial for pathogens like viruses, bacteria, or parasites. For example, methotrexate is used in chemotherapy to inhibit enzymes involved in DNA synthesis, while protease inhibitors are employed to treat HIV/AIDS by inhibiting the virus’s protease enzyme.
- In conclusion, enzyme inhibition is a complex yet essential process that plays a pivotal role in various biological and medical contexts. Through detailed and sequential explanations, it becomes evident that enzyme inhibitors, whether naturally occurring or synthetically designed, have profound implications for cellular function, ecological interactions, and human health.
Types of Enzyme Inhibition
There are present three types of Enzyme Inhibition such as;
- Reversible Inhibition:
- Definition: Reversible inhibition refers to the temporary binding of an inhibitor to an enzyme, which results in a reduction of the enzyme’s catalytic activity. This type of inhibition is not permanent, and the enzyme can regain its activity once the inhibitor is removed.
- Function: The primary function of reversible inhibitors is to modulate enzyme activity based on cellular needs. They can be quickly added or removed, allowing for rapid adjustments in metabolic pathways.
- Mechanism: Reversible inhibitors bind non-covalently to the enzyme. They can either compete with the substrate for the enzyme’s active site (competitive inhibition) or bind to a different site on the enzyme, altering its conformation and affecting its ability to bind the substrate or catalyze the reaction (non-competitive inhibition).
- Irreversible Inhibition:
- Definition: Irreversible inhibition involves the permanent binding of an inhibitor to an enzyme, rendering the enzyme inactive for an extended period or permanently.
- Function: Irreversible inhibitors can serve as regulatory agents in specific metabolic pathways or act as protective agents against harmful enzymes. They can also be used therapeutically to target aberrant enzymes or pathogens.
- Mechanism: These inhibitors form a strong covalent bond with the enzyme, leading to long-lasting or permanent inactivation. The bond can only be broken under specific conditions, if at all.
- Allosteric Inhibition:
- Definition: Allosteric inhibition is a specialized form of enzyme regulation where the inhibitor binds to a site other than the enzyme’s active site, known as the allosteric site.
- Function: Allosteric inhibitors play a crucial role in maintaining cellular homeostasis by regulating enzyme activity in response to cellular signals or feedback mechanisms.
- Mechanism: When an allosteric inhibitor binds to the enzyme, it induces a conformational change in the enzyme’s structure. This change can either enhance or reduce the enzyme’s affinity for its substrate, thereby modulating its activity.
1. Reversible Inhibition
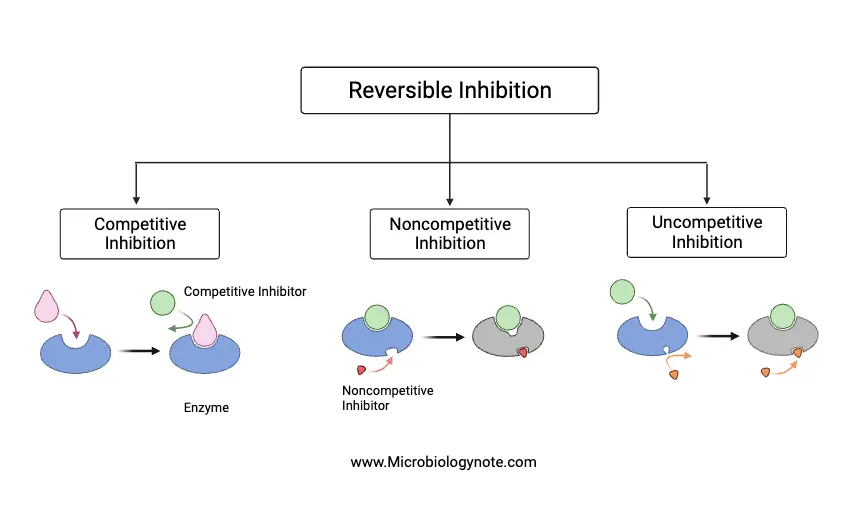
Reversible inhibition refers to the temporary cessation of enzyme activity due to the binding of a reversible inhibitor. This binding is not permanent, and the enzyme can regain its activity once the inhibitor is removed. The hallmark of reversible inhibition is the non-covalent interactions, such as hydrogen bonding and hydrophobic interactions, that facilitate the binding of the inhibitor to the enzyme. Despite being weak individually, these interactions collectively result in strong binding. Importantly, these inhibitors do not undergo any chemical reactions with the enzyme, ensuring that their inhibitory effect can be easily reversed by dilution or dialysis.
Types of Reversible Inhibition
Reversible inhibition can be categorized into three primary types based on specific criteria:
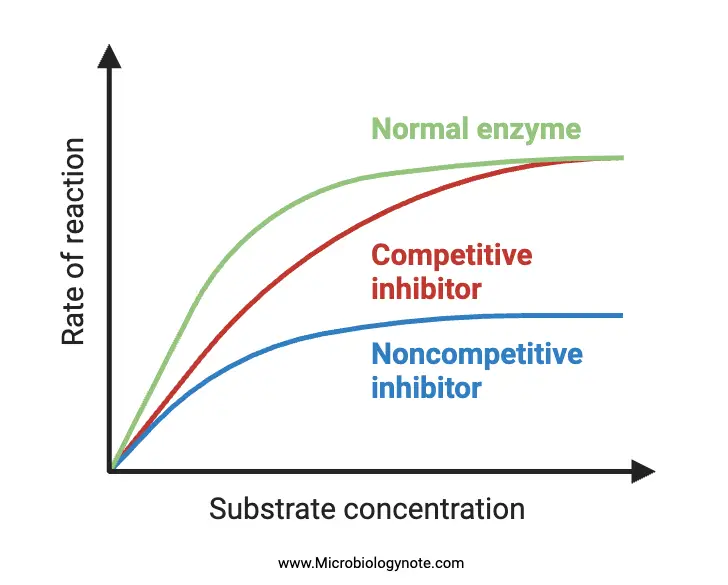
- Competitive Inhibition:
- Definition: Competitive inhibitors compete with the substrate for binding to the enzyme’s active site.
- Function: By binding to the active site, these inhibitors prevent the substrate from accessing the enzyme, thereby inhibiting the reaction.
- Mechanism: The inhibitory effect of competitive inhibitors can be overcome by increasing the substrate concentration. This is because, with a higher substrate concentration, the likelihood of the substrate binding to the enzyme increases, outcompeting the inhibitor.
- Noncompetitive Inhibition:
- Definition: Noncompetitive inhibitors bind to an allosteric site, which is distinct from the enzyme’s active site.
- Function: The binding of the inhibitor induces a conformational change in the enzyme, rendering it less effective or inactive.
- Mechanism: The presence of the substrate does not influence the binding of noncompetitive inhibitors. Therefore, increasing substrate concentration does not negate the inhibitory effect.
- Uncompetitive Inhibition:
- Definition: Uncompetitive inhibitors bind only to the enzyme-substrate complex.
- Function: This type of inhibition reduces the enzyme’s turnover rate by preventing the complex from releasing products.
- Mechanism: The inhibitor binds at a site distinct from the active site, and its binding is enhanced when the substrate is present.
a. Competitive inhibition
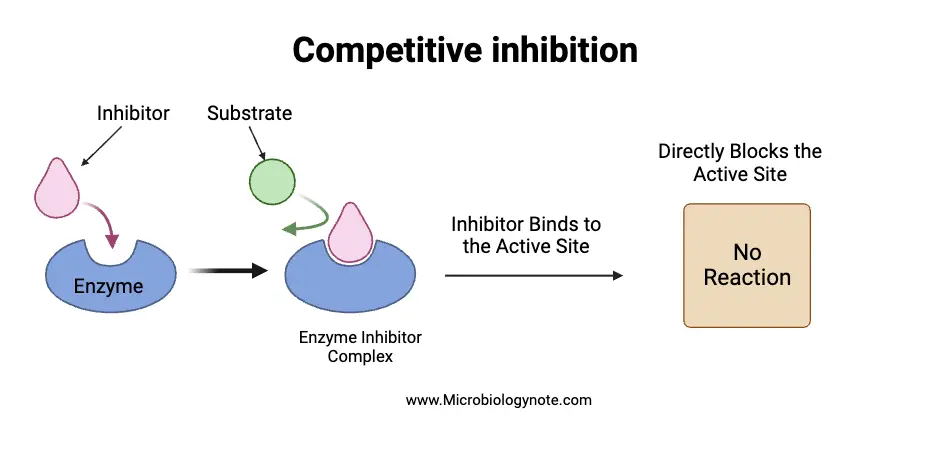
Competitive inhibition refers to the phenomenon where inhibitors compete with the substrate for binding to the enzyme’s active site. This competition arises because the competitive inhibitor often bears a close structural resemblance to the substrate. As a result, the enzyme may form an enzyme-inhibitor (EI) complex instead of the desired enzyme-substrate (ES) complex. The degree of inhibition is directly influenced by the concentrations of both the substrate and the inhibitor. Therefore, the inhibitory effect can be intensified by increasing the inhibitor concentration or mitigated by elevating the substrate concentration.
- Mechanisms and Dynamics: The competitive inhibitor, often regarded as a substrate analogue, binds to the enzyme’s active site but does not undergo catalysis. This binding effectively blocks the enzyme’s active site, rendering it unavailable for the substrate. The formation of both ES and EI complexes occurs simultaneously, with the relative concentrations of substrate and inhibitor, along with their affinities for the enzyme, determining the extent of competitive inhibition. Notably, at high substrate concentrations, the inhibitory effects of a competitive inhibitor can be reversed, as the substrate can effectively outcompete the inhibitor for binding to the active site.
- Functional Implications: A competitive inhibitor reduces the rate of enzymatic reactions by decreasing the proportion of enzyme molecules bound to a substrate. However, it’s crucial to note that while the Km value (substrate concentration at which the reaction rate is half of Vmax) increases in the presence of a competitive inhibitor, the Vmax (maximum reaction rate) remains unchanged.
- Notable Examples:
- Succinate Dehydrogenase (SDH): This enzyme, which uses succinic acid as its substrate, is a classic example of competitive inhibition. Compounds like malonic acid, glutaric acid, and oxalic acid, due to their structural similarity to succinic acid, can act as competitive inhibitors, binding to SDH’s active site.
- Methanol Poisoning: Methanol becomes toxic when converted to formaldehyde by the enzyme alcohol dehydrogenase (ADH). Ethanol, due to its competitive nature, can bind to ADH, preventing methanol conversion and serving as a treatment for methanol poisoning.
- Antimetabolites: These are chemical compounds that obstruct metabolic reactions through their inhibitory action on enzymes. Being structural analogues of substrates, they function as competitive inhibitors and have found applications in cancer therapy and treating conditions like gout. Some antimetabolites even block the biochemical actions of vitamins, leading to deficiencies.
b. Noncompetitive inhibition
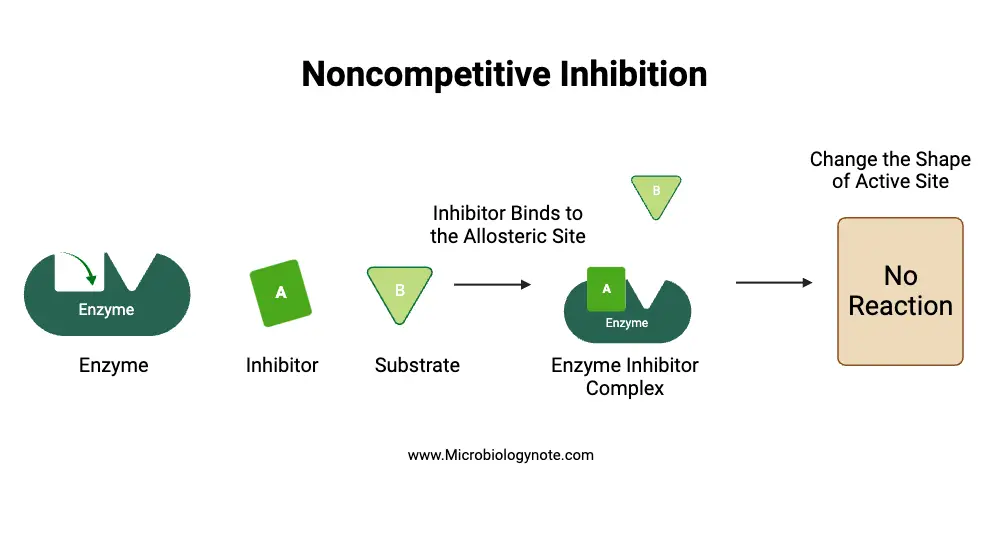
Noncompetitive inhibition refers to the scenario where enzymatic activity is hindered by inhibitors binding to a location on the enzyme other than the active site. The term “noncompetitive” indicates the absence of competition between the substrate and the inhibitor for the active site. Furthermore, these inhibitors, known as noncompetitive inhibitors, do not bear any structural resemblance to the substrate. This unique binding mechanism allows for the simultaneous formation of both the enzyme-inhibitor complex and the enzyme-substrate complex.
- Mechanisms and Dynamics: In noncompetitive inhibition, both the inhibitor and substrate can concurrently bind to the same enzyme molecule, given that their binding sites are distinct and do not overlap. The binding of the inhibitor induces conformational changes in the enzyme’s active site, thereby preventing the substrate from binding effectively. Besides, this binding process effectively diminishes the concentration of active enzymes, leading to a reduced reaction rate. Notably, unlike competitive inhibition, the inhibitory effects of noncompetitive inhibition cannot be reversed by simply increasing the substrate concentration.
- Functional Implications: The primary consequence of noncompetitive inhibition is the enzyme’s inactivation when an inhibitor is bound, irrespective of the presence of the substrate. This form of inhibition is particularly potent as it results in the enzyme’s functional impairment, even when the substrate is present in abundance. The binding of noncompetitive inhibitors often leads to significant alterations in the enzyme’s conformation, rendering it inactive.
- Notable Examples: Heavy metal ions, such as Ag+, Hg2+, and Pb2+, serve as classic examples of noncompetitive inhibitors. These ions can inhibit a plethora of enzymes, like urease. The mechanism involves these heavy metal ions binding to specific sites on the enzyme, often leading to irreversible inhibition. For instance, Hg2+ can bind with cysteinyl sulfhydryl groups, resulting in noncompetitive inhibition. Additionally, heavy metals can form covalent bonds with carboxyl groups and histidine, further emphasizing their inhibitory potency.
c. Uncompetitive inhibition
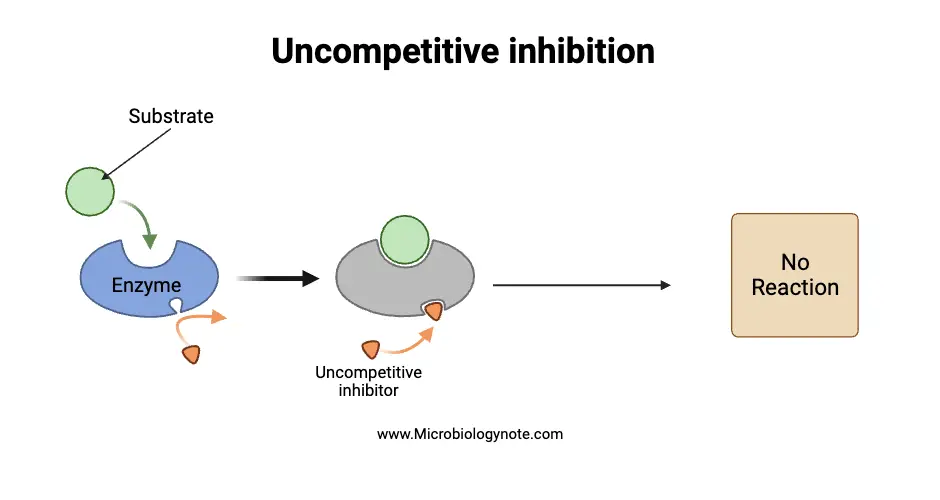
Uncompetitive inhibition refers to a specific type of enzymatic activity regulation where the inhibitor binds to an allosteric site on the enzyme. However, what sets it apart is that this binding occurs exclusively with the enzyme-substrate (ES) complex and not with the free enzyme molecule. This specificity in binding ensures that the inhibitor only interacts when the enzyme has already bound to its substrate.
- Mechanistic Insights: The primary mechanism underlying uncompetitive inhibition revolves around the removal of the activated enzyme-substrate complex from the reaction milieu. By binding to this complex, the uncompetitive inhibitor effectively reduces its availability, leading to a decrease in the maximum velocity (Vmax) of the associated chemical reaction. This means that even if the substrate concentration is increased, the reaction rate will not reach its previous maximum potential due to the inhibitor’s presence.
- Functional Implications: The binding of the uncompetitive inhibitor to the ES complex has profound implications for enzymatic reactions. Since the inhibitor specifically targets the activated ES complex, it ensures that the reaction’s rate is modulated at a stage where the substrate has already interacted with the enzyme. This form of inhibition is particularly effective in scenarios where it’s essential to control reactions post-substrate binding.
- Noteworthy Examples: To understand the real-world significance of uncompetitive inhibition, one can look at specific examples. For instance, compounds like tetramethylene sulfoxide and 3-butylthiolene oxide act as uncompetitive inhibitors for the enzyme liver alcohol dehydrogenase. Their presence ensures that the enzymatic activity of liver alcohol dehydrogenase is modulated, emphasizing the practical relevance of uncompetitive inhibition in biological systems.
D. Mixed inhibition
Mixed inhibition is a type of enzyme inhibition where the inhibitor can bind to the enzyme regardless of whether the substrate has already attached to the enzyme. This form of inhibition is essentially a blend of both competitive and noncompetitive inhibition. In this context, it’s crucial to understand that the inhibitor’s affinity might vary when binding to the free enzyme compared to when it binds to the enzyme-substrate complex.
In the realm of biochemistry, the implications of mixed inhibition are profound. As the substrate concentration ([S]) increases, the inhibitory effect can be diminished due to the competitive aspect of this inhibition. However, it cannot be completely nullified because of the noncompetitive component.
A distinctive feature of mixed inhibition is that, although it’s feasible for mixed-type inhibitors to attach within the active site, this inhibition typically arises from an allosteric effect. This means the inhibitor binds to a site on the enzyme that is different from the active site. When the inhibitor attaches to this allosteric site, it induces a conformational change in the enzyme. This alteration in the enzyme’s tertiary structure or three-dimensional shape leads to a decreased affinity of the substrate for the active site.
Furthermore, it’s essential to differentiate between the four types of inhibition based on the impact of increasing the substrate concentration [S] on the degree of inhibition caused by a specific amount of inhibitor. For instance:
- In competitive inhibition, the degree of inhibition lessens with an increase in [S].
- In noncompetitive inhibition, the degree of inhibition remains constant regardless of [S].
- In uncompetitive (or anticompetitive) inhibition, the degree of inhibition amplifies with an increase in [S].
Therefore, understanding mixed inhibition is pivotal in the study of enzyme kinetics, as it provides insights into the intricate interactions between enzymes, substrates, and inhibitors.
2. Irreversible inhibition
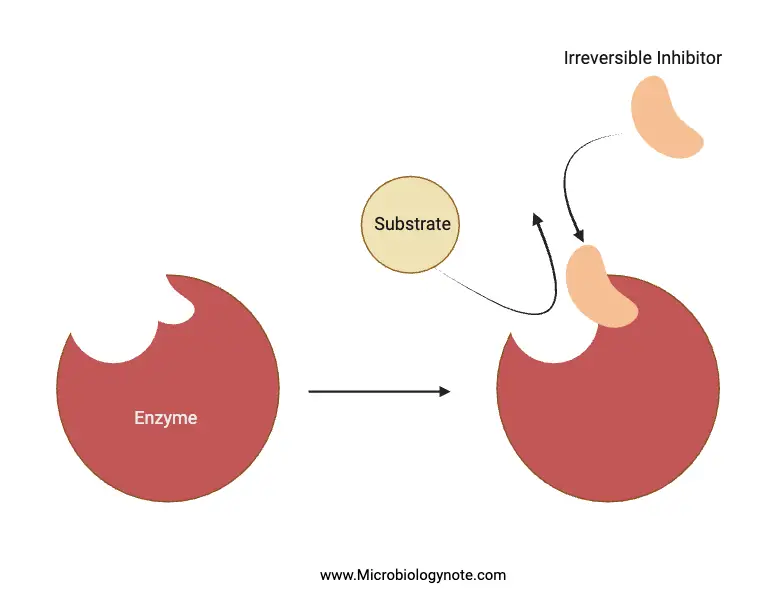
Irreversible inhibition refers to the cessation of enzymatic activity resulting from permanent structural changes induced by the binding of an inhibitor. This binding often leads to the destruction of essential functional groups on the enzyme, crucial for its activity. Unlike reversible inhibition, which can be reversed by removing the inhibitor, irreversible inhibition is enduring and cannot be undone.
Mechanistic Insights: The binding of irreversible inhibitors is characterized by strong covalent bonds, often facilitated by the inhibitor’s reactive functional groups such as aldehydes, alkenes, and haloalkanes. This covalent bonding ensures that the inhibitor remains tightly bound to the enzyme’s active site, leading to its inactivation. Furthermore, irreversible inhibitors tend to be highly specific, targeting a particular group of enzymes. They achieve enzyme inactivation by altering the active site rather than destroying the protein’s overall structure.
Functional Implications: The impact of irreversible inhibition on enzymatic activity is time-dependent, typically manifesting in an exponential manner. Given the permanent nature of this inhibition, the affected enzymes are rendered inactive for extended periods, potentially leading to significant physiological implications.
Examples: To comprehend the significance of irreversible inhibition, one can consider specific examples:
- Alkylating Agents: Compounds like iodoacetamide serve as irreversible inhibitors, targeting enzymes by modifying functional groups such as cysteine side chains.
- Nerve Gases: Diisopropyl fluorophosphate (DFP), developed during the Second World War, binds irreversibly to enzymes with serine at their active sites, including vital enzymes like acetylcholine esterase.
- Insecticides: Organophosphorus insecticides, such as melathion, can be toxic as they block acetylcholine esterase, crucial for nerve conduction, leading to paralysis.
- Medications: Disulfiram, used in treating alcoholism, irreversibly inhibits aldehyde dehydrogenase, causing acetaldehyde accumulation and promoting alcohol avoidance.
- Antibiotics: Penicillin antibiotics act as irreversible inhibitors of serine-containing enzymes, hindering bacterial cell wall synthesis.
Suicide inhibition
Suicide inhibition, also known as mechanism-based inhibition, is a subtype of irreversible inhibition. What sets it apart is the intriguing process wherein the original inhibitor, which is typically a structural analogue or competitive inhibitor, undergoes transformation by the very enzyme it aims to inhibit. This transformation results in the formation of a more potent inhibitor, which then binds irreversibly to the enzyme. This is in stark contrast to the initial inhibitor, which binds in a reversible manner.
Mechanistic Insights: The essence of suicide inhibition lies in its self-destructive nature. The enzyme, in its attempt to catalyze the inhibitor, inadvertently converts it into a more potent form. This newly formed inhibitor then binds permanently to the enzyme, rendering it inactive. Therefore, the enzyme, in essence, participates in its own inactivation.
Notable Examples: To truly grasp the significance of suicide inhibition, it’s beneficial to consider specific instances:
- Allopurinol: A prime example of suicide inhibition is the action of allopurinol, commonly used in treating gout. Allopurinol, an inhibitor of the enzyme xanthine oxidase, undergoes transformation to become alloxanthine. This converted form, alloxanthine, is a more effective inhibitor of xanthine oxidase, showcasing the classic mechanism of suicide inhibition.
- Cancer Therapy Analogues: The principle of suicide inhibition finds application in cancer therapy. Certain purine and pyrimidine analogues, used in cancer treatments, operate based on this mechanism. For instance, 5-fluorouracil is transformed into fluorodeoxyuridylate, which subsequently inhibits the enzyme thymidylate synthase. This inhibition disrupts nucleotide synthesis, a crucial process for cancer cell proliferation.
In conclusion, suicide inhibition stands out as a unique regulatory mechanism in enzymology, with profound therapeutic implications. Through a methodical, objective, and technical exposition, we unravel the complexities of suicide inhibition, offering a holistic understanding of this fascinating enzymatic phenomenon.
3. Allosteric inhibition
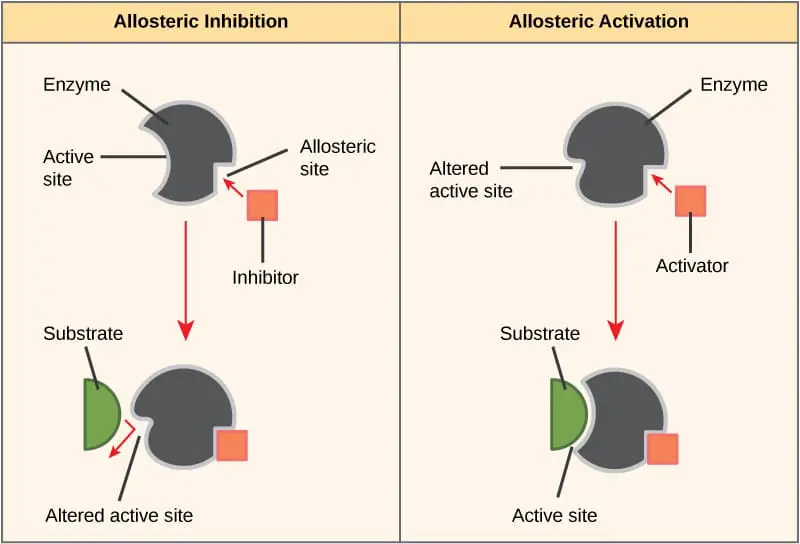
Allosteric inhibition is a type of enzyme regulation wherein the binding of an inhibitor to an enzyme’s allosteric site induces a conformational change. This change, distinct from the enzyme’s active site, results in a decrease in the enzyme’s activity. It’s essential to note that the inhibitor does not bind to the active site, but rather to a separate, specific site, known as the allosteric site.
- Mechanistic Overview: The core of allosteric inhibition lies in its ability to modulate enzyme activity without directly interfering with the substrate binding. When an allosteric inhibitor binds to the enzyme, it induces a structural shift in the enzyme’s conformation. This shift, in turn, affects the enzyme’s active site, rendering it less receptive to its substrate. Therefore, the overall enzymatic activity diminishes.
- Role in Living Systems: In living organisms, enzymes play a crucial role in catalyzing myriad biochemical reactions. To ensure that these reactions occur at the right time and pace, regulatory mechanisms like allosteric inhibition are indispensable. By modulating enzyme activity, allosteric inhibition ensures that metabolic pathways are finely tuned, maintaining the delicate balance of biological processes.
- Significance of Allosteric Regulation: Allosteric inhibition is a subset of the broader concept of allosteric regulation. This regulation encompasses both inhibition and activation, offering a dynamic range of control over enzyme activity. In essence, allosteric regulation serves as a feedback mechanism, ensuring that enzymes respond aptly to the needs of the cell.
Applications of Enzyme Inhibition
- Therapeutic Drug Development: In the realm of medicine, enzyme inhibitors serve as the foundation for numerous therapeutic drugs. By targeting specific enzymes associated with disease pathways, these drugs can alleviate symptoms, halt disease progression, or even cure certain conditions. For instance, ACE inhibitors are used to treat hypertension by inhibiting the angiotensin-converting enzyme, thereby reducing blood pressure.
- Agriculture and Pest Control: In the agricultural sector, enzyme inhibitors play a pivotal role in pest management. Insecticides, such as malathion, function by inhibiting essential enzymes in pests, leading to their elimination. Similarly, herbicides like glyphosate inhibit enzymes in weeds, ensuring crop protection and enhanced yield.
- Disinfectants and Antimicrobial Agents: Certain enzyme inhibitors, like triclosan, are employed as disinfectants. By inhibiting bacterial enzymes, these agents prevent bacterial growth, ensuring hygiene and public health. Their use is widespread in hand sanitizers, soaps, and other cleaning agents.
- Neurological Applications: Enzyme inhibitors have a significant role in neurology, especially in the treatment of neurodegenerative disorders. For instance, cholinesterase inhibitors are used in the management of Alzheimer’s disease to enhance neurotransmission in the brain.
- Cancer Treatment: In the fight against cancer, enzyme inhibitors have emerged as potent allies. By targeting enzymes that facilitate cancer cell growth and proliferation, these inhibitors can halt tumor progression. Drugs like tyrosine kinase inhibitors are used in the treatment of various cancers.
- Research and Diagnostic Tools: In the world of research, enzyme inhibitors are invaluable tools. They help in understanding enzyme functions, pathways, and their implications in health and disease. Additionally, they are used in diagnostic assays to detect specific conditions or pathogens.
Differences Between Competitive, Uncompetitive, and Noncompetitive Inhibition
Competitive Inhibition:
- In competitive inhibition, the inhibitor competes with the substrate for binding at the enzyme’s active site. This type of inhibition is also referred to as Substrate Analogue Inhibition.
- A complex known as the enzyme-inhibitor complex [EI] is formed, which is similar to the enzyme-substrate complex [ES].
- The extent of inhibition is influenced by the relative concentrations of both the substrate and the inhibitor. As the substrate concentration increases, the rate of inhibition can decrease.
- An example of this type of inhibition involves the enzyme Succinate Dehydrogenase, the substrate Succinate, and inhibitors such as Malonate, Glutarate, and Oxalate.
- Kinetics of competitive inhibition show a change in the slope [Km/Vmax], but the intercept on the ordinate [1/Vmax] remains unchanged.
Uncompetitive Inhibition:
- In uncompetitive inhibition, the inhibitor does not bind with the free enzyme. Instead, it binds with the enzyme-substrate complex.
- An inactive enzyme-substrate-inhibitor complex [ESI] is formed, preventing the formation of the product.
- Interestingly, increasing the substrate concentration might enhance the degree of inhibition in this type.
- This form of inhibition is rare in reactions involving a single substrate but is common in bi-substrate reactions.
- Kinetically, the slope [Km/Vmax] remains consistent, but there’s a change in the intercept on the ordinate [1/Vmax].
Noncompetitive Inhibition:
- Noncompetitive inhibitors can bind to either the free enzyme or the enzyme-substrate complex, affecting both their functions. These inhibitors attach to a site on the enzyme that’s different from the active site.
- Such inhibitors often deform the enzyme, leading to a reduced rate of [ES] complex formation and decomposition. Two inactive complexes, [ESI] and [EI], are formed as a result.
- Notably, increasing the substrate concentration does not reverse the degree of inhibition in noncompetitive inhibition.
- An example includes enzymes that require metal ions, which can be inhibited by chelating agents like EDTA.
- In terms of kinetics, both the slope [Km/Vmax] and the intercept on the ordinate [1/Vmax] undergo changes.
Type of Inhibition | Competitive | Uncompetitive | Noncompetitive |
---|---|---|---|
Description | The inhibitor competes with the substrate for binding at the enzyme’s active site. | The inhibitor binds with the enzyme-substrate complex. | Inhibitors can bind to either the free enzyme or the enzyme-substrate complex. |
Complex Formed | Enzyme-inhibitor complex [EI] | Inactive enzyme-substrate-inhibitor complex [ESI] | Two inactive complexes: [ESI] and [EI] |
Effect of Increasing Substrate Concentration | Decreases rate of inhibition | Increases degree of inhibition | No reversal of inhibition |
Examples | Enzyme: Succinate Dehydrogenase; Substrate: Succinate; Inhibitors: Malonate, Glutarate, Oxalate | Rare in single-substrate reactions; common in bi-substrate reactions | Enzymes requiring metal ions; inhibited by chelating agents like EDTA |
Kinetics | Change in slope [Km/Vmax]; No change in intercept [1/Vmax] | No change in slope [Km/Vmax]; Change in intercept [1/Vmax] | Change in both slope [Km/Vmax] and intercept [1/Vmax] |