Table of Contents
What is Receptor tyrosine kinases (RTKs)?
- Receptor tyrosine kinases (RTKs) constitute a crucial category of high-affinity cell surface receptors, playing pivotal roles in mediating cellular responses to various extracellular signals such as polypeptide growth factors, cytokines, and hormones. Within the human genome, 58 out of 90 unique tyrosine kinase genes encode RTK proteins, underscoring their significance in cellular regulation and communication processes. The relevance of RTKs extends beyond normal cellular functions, as their involvement in the development and progression of diverse cancers has been well-established.
- These receptors belong to the broader family of protein tyrosine kinases, distinguishing them from non-receptor tyrosine kinases by the presence of a transmembrane domain. The first RTKs discovered were the EGF and NGF receptors in the 1960s, with the classification of RTKs emerging in the subsequent decade. Tyrosine phosphorylation, a critical post-translational modification for inter- and intracellular communication, is facilitated by the activity of RTKs among other protein tyrosine kinases.
- RTKs operate as single-pass, type I receptors embedded in the plasma membrane, typically activated through ligand-induced oligomerization, notably dimerization. This activation leads to autophosphorylation of tyrosine residues, triggering downstream signaling cascades. The spatial and temporal aspects of cellular signal propagation have become more accessible through advances in proteomics and live-cell imaging, enhancing our understanding of the intricate mechanisms governing RTK activation.
- In the context of cell-to-cell communication, RTKs serve as essential components of signal transduction pathways. Ligand binding to the extracellular domain of RTKs initiates a cascade of events, including intracellular activation of the kinase domains. This activation prompts conformational changes, stabilizing the active state of the kinase, and providing docking sites for downstream signaling proteins. The structural and mechanistic details of ligand-induced RTK oligomerization remain active areas of research, reflecting the complexity of these processes.
- Cells, to fulfill their physiological role, rely on the reception, integration, and response to extracellular messengers. The receptors, including RTKs, are vital for this communication, typically located at the cell membrane. The ligand-receptor binding is specific, reversible, and involves various low-energy bonds, ensuring equilibrium.
- The dysregulation of ligand-receptor systems, such as RTKs, can lead to pathological situations, including cancer. In the context of bone cancers and metastases, dysregulated RTKs contribute to disturbances in cell communication, leading to uncontrolled bone resorption and subsequent cancer cell proliferation.
- To address these issues, chemical drugs have been developed to target specific RTKs activated by mutations or ligands in the tumor microenvironment. These drugs aim to restore balance and regulate the aberrant signaling associated with RTK dysregulation, particularly in the treatment of bone cancers and metastases.
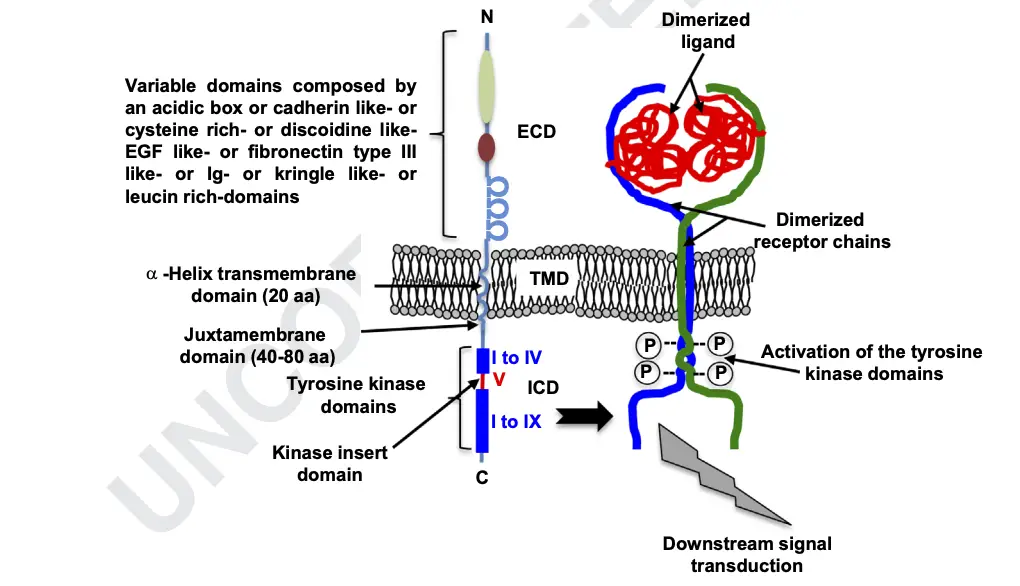
Characteristics of Receptor tyrosine kinases (RTKs)
Receptor tyrosine kinases (RTKs) exhibit distinctive characteristics that define their functional roles in cellular processes and their significance in various physiological and pathological conditions. The following outlines key characteristics of RTKs:
- Cell Surface Receptors:
- RTKs are located on the cell surface, embedded in the plasma membrane. They are single-pass transmembrane proteins, ensuring their exposure to the extracellular environment.
- Activation through Ligand Binding:
- RTKs are activated by binding to specific ligands, which are often polypeptide growth factors, cytokines, or hormones. Ligand binding induces conformational changes in the receptor, initiating downstream signaling.
- High-Affinity Binding:
- RTKs exhibit high-affinity binding with their ligands. This specificity ensures precise cellular responses to particular signaling molecules, contributing to the regulation of various cellular functions.
- Tyrosine Kinase Activity:
- A defining feature of RTKs is their intrinsic enzymatic activity as tyrosine kinases. The cytoplasmic domain of RTKs possesses kinase activity, allowing them to phosphorylate tyrosine residues on themselves (autophosphorylation) and on downstream signaling proteins.
- Oligomerization upon Activation:
- RTKs are often activated through ligand-induced oligomerization. Ligand binding leads to receptor dimerization or higher-order oligomerization, bringing the kinase domains in close proximity and facilitating autophosphorylation.
- Downstream Signaling Pathways:
- Phosphorylated tyrosine residues on activated RTKs serve as docking sites for downstream signaling proteins. These interactions initiate signaling cascades that regulate cellular processes such as growth, differentiation, metabolism, and motility.
- Involvement in Cancer Pathogenesis:
- Mutations in RTKs are associated with the development and progression of various types of cancer. Gain-of-function mutations or overexpression of RTKs can lead to uncontrolled cell growth and survival, contributing to tumorigenesis.
- Critical Role in Development:
- RTKs play a crucial role in embryonic development and adult tissue homeostasis. They are involved in processes like organogenesis and cellular differentiation, highlighting their significance in overall developmental biology.
- Specificity in Ligand Recognition:
- Ligand-receptor interactions are highly specific, involving multiple low-energy bonds such as hydrogen, ionic, hydrophobic, and Van der Waals forces. This specificity ensures that each RTK responds to its particular ligand.
- Regulation of Tissue Homeostasis:
- RTKs tightly control tissue homeostasis, and any dysregulation, whether through mutations or overexpression, can disrupt normal cell communication and lead to pathological conditions.
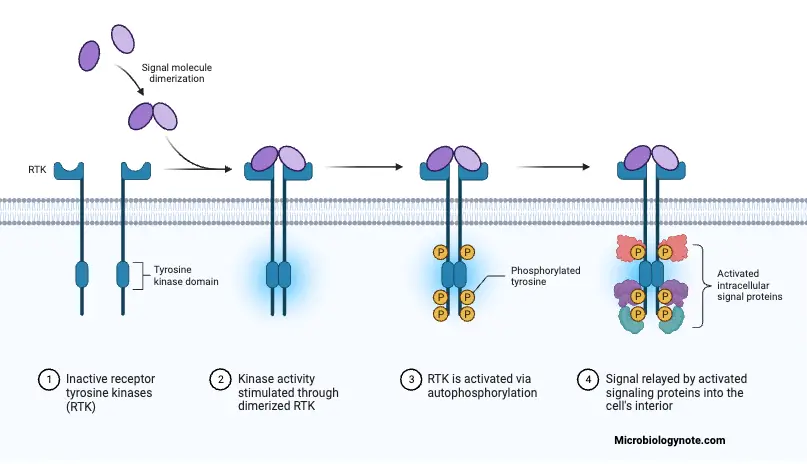
Structure of Receptor tyrosine kinases (RTKs)
- Monomeric and Multimeric Structure:
- Most RTKs are characterized as single subunit receptors; however, some, like the insulin receptor, exist as multimeric complexes. The insulin receptor forms disulfide-linked dimers upon the presence of insulin hormone. Ligand binding induces the formation of receptor dimers.
- Subunit Composition:
- Each RTK monomer consists of a single hydrophobic transmembrane-spanning domain, typically composed of 25 to 38 amino acids. The receptor structure includes an extracellular N-terminal region and an intracellular C-terminal region.
- Extracellular Domain Characteristics:
- The extracellular N-terminal region displays conserved elements such as immunoglobulin (Ig)-like or epidermal growth factor (EGF)-like domains, fibronectin type III repeats, or cysteine-rich regions specific to each RTK subfamily. These domains primarily house ligand-binding sites that interact with extracellular ligands, such as growth factors or hormones.
- Intracellular C-Terminal Region:
- The intracellular C-terminal region exhibits a high level of conservation and contains catalytic domains responsible for the kinase activity of RTKs. This kinase activity facilitates receptor autophosphorylation and tyrosine phosphorylation of downstream substrates.
- Protein Kinase Activity:
- RTKs, as a subset of protein kinases, play a key role in cellular regulation by catalyzing the transfer of phosphate groups. Among the 90 genes encoding proteins with tyrosine kinase activity, 58 encode RTKs divided into 20 subfamilies.
- Transmembrane Domain and Glycosylation:
- RTKs are inserted into the cell membrane through a transmembrane domain composed of 20 amino acids. This domain plays a crucial role in receptor chain dimerization and stabilization. The N-terminal extracellular domain is glycosylated and involved in ligand recognition.
- Tyrosine Kinase Domain Structure:
- The cytoplasmic domain houses the tyrosine kinase activity responsible for phosphorylating receptor chains. It includes a juxtamembrane region and a carboxyterminal region. The tyrosine kinase domain, composed of 12 subdomains, has an activation loop determining the active or inactive state of the kinase domain
- Structural Variability Between RTK Families:
- While the size of the tyrosine kinase domain remains relatively constant among different RTKs, the juxta- and C-terminal domains exhibit considerable variability between RTK families. This variability confers specificity to intracellular signals and contributes to the diversity of RTK functions.
- Tyrosine Residue Distribution:
- The number and distribution of tyrosine residues vary significantly among RTKs. Activated tyrosine residues, particularly in the activation loop, are crucial for maintaining the “open” conformation of both lobes and facilitating ATP and substrate binding.
- Functional Implications of Tyrosine Residues:
- Tyrosine residues, especially those phosphorylated after RTK activation, stabilize the conformation of the activation loop and both lobes. Mutations in these residues result in the inactivation of receptor chains. Notably, EGFR is an exception in the RTK families, possessing a unique structural configuration.
Classes of Receptor tyrosine kinases (RTKs)
Approximately 20 distinct classes of Receptor Tyrosine Kinases (RTKs) have been identified, each playing unique roles in cellular signaling and regulation.
- Class I – EGF Receptor Family (ErbB Family):
- Includes epidermal growth factor receptor (EGFR) family or ErbB family. Critical for cellular signaling, with aberrant signaling linked to various diseases, including cancer.
- Class II – Insulin Receptor Family:
- Constitutes insulin receptor family, essential for insulin signaling and central in regulating glucose metabolism.
- Class III – PDGF Receptor Family:
- Encompasses platelet-derived growth factor (PDGF) receptor family, involved in cellular processes like growth and development.
- Class IV – VEGF Receptors Family:
- Includes vascular endothelial growth factor (VEGF) receptor family, crucial for endothelial cell proliferation and blood vessel permeability, contributing to angiogenesis.
- Class V – FGF Receptor Family:
- Constitutes fibroblast growth factor (FGF) receptor family, essential for processes such as cell proliferation, differentiation, and tissue repair.
- Class VI – CCK Receptor Family:
- Encompasses the cholecystokinin (CCK) receptor family, mediating signals related to gastrointestinal functions.
- Class VII – NGF Receptor Family:
- Falls under the nerve growth factor (NGF) receptor family, crucial for the development and maintenance of the nervous system.
- Class VIII – HGF Receptor Family:
- Encompasses hepatocyte growth factor (HGF) receptor family, involved in processes such as cell motility, morphogenesis, and tissue repair.
- Class IX – Eph Receptor Family:
- Falls under the Eph receptor family, critical for cellular processes including cell adhesion, repulsion, and axon guidance.
- Class X – AXL Receptor Family:
- Consists of the AXL receptor family, playing roles in immune regulation, cell survival, and cancer progression.
- Class XI – TIE Receptor Family:
- Categorized as the TIE receptor family, involved in angiogenesis and vascular development.
- Class XII – RYK Receptor Family:
- Encompasses the RYK receptor family, implicated in processes related to neuronal development and axon guidance.
- Class XIII – DDR Receptor Family:
- Falls under the Discoidin Domain Receptor (DDR) family, uniquely binding to collagens and mediating cellular responses in the extracellular matrix.
- Class XIV – RET Receptor Family:
- Includes the RET receptor family, responding to glial cell line-derived neurotrophic factor (GDNF) family ligands and playing roles in neuronal development.
- Class XV – ROS Receptor Family:
- Categorized as the ROS receptor family, involved in redox signaling and regulation of cellular responses to oxidative stress.
- Class XVI – LTK Receptor Family:
- Consists of the leukocyte tyrosine kinase (LTK) receptor family, implicated in immune regulation and potential roles in hematopoiesis.
- Class XVII – ROR Receptor Family:
- Encompasses the ROR receptor family, including receptors playing roles in development, immunity, and circadian rhythm regulation.
- Class XVIII – MuSK Receptor Family:
- Includes the muscle-specific kinase (MuSK) receptor family, crucial for neuromuscular regulation.
- Class XIX – LMR Receptor:
- Represents a distinct class associated with specific cellular functions or yet to be fully characterized.
- Class XX – Undetermined:
- Represents an undetermined class requiring further characterization and classification.
Class | Receptor Family | Function |
---|---|---|
I | EGF Receptor Family (ErbB Family) | Cellular signaling, implicated in diseases like cancer |
II | Insulin Receptor Family | Regulates glucose metabolism |
III | PDGF Receptor Family | Involved in cellular processes such as growth and development |
IV | VEGF Receptors Family | Critical for endothelial cell proliferation and blood vessel permeability |
V | FGF Receptor Family | Essential for cell proliferation, differentiation, and tissue repair |
VI | CCK Receptor Family | Mediates signals related to gastrointestinal functions |
VII | NGF Receptor Family | Crucial for the development and maintenance of the nervous system |
VIII | HGF Receptor Family | Involved in cell motility, morphogenesis, and tissue repair |
IX | Eph Receptor Family | Critical for cell adhesion, repulsion, and axon guidance |
X | AXL Receptor Family | Roles in immune regulation, cell survival, and cancer progression |
XI | TIE Receptor Family | Involved in angiogenesis and vascular development |
XII | RYK Receptor Family | Implicated in neuronal development and axon guidance |
XIII | DDR Receptor Family | Unique binding to collagens, mediating cellular responses in the extracellular matrix |
XIV | RET Receptor Family | Responds to GDNF family ligands, roles in neuronal development |
XV | ROS Receptor Family | Involved in redox signaling and regulation of cellular responses to oxidative stress |
XVI | LTK Receptor Family | Implicated in immune regulation and potential roles in hematopoiesis |
XVII | ROR Receptor Family | Roles in development, immunity, and circadian rhythm regulation |
XVIII | MuSK Receptor Family | Crucial for neuromuscular regulation |
XIX | LMR Receptor | Associated with specific cellular functions or yet to be fully characterized |
XX | Undetermined | Represents a class requiring further characterization and classification |
Families of Receptor tyrosine kinases (RTKs)
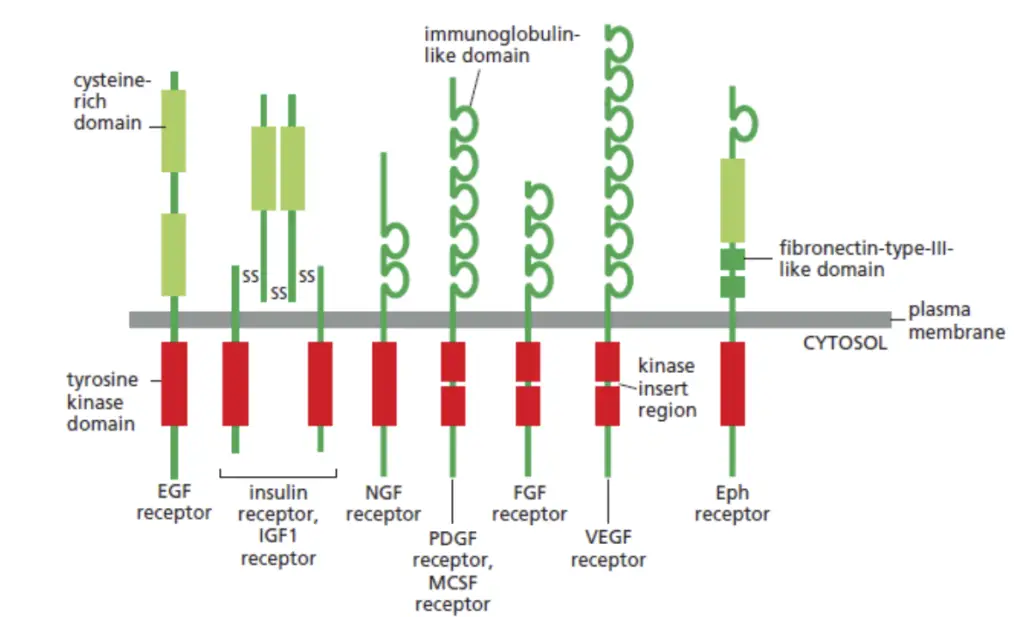
The Receptor Tyrosine Kinase (RTK) family comprises a diverse array of members, each intricately involved in regulating specific cellular signaling pathways. These receptors, characterized by their ability to phosphorylate tyrosine residues, play pivotal roles in various physiological processes. Notable members of the RTK family include:
- Epidermal Growth Factor Receptor (EGFR or ErbB1):
- Function: Associated with cell growth, development, and differentiation.
- Implications: Implicated in cancer, notably promoting tumor growth.
- Clinical Correlations: Dysregulation linked to conditions like multiple sclerosis and Alzheimer’s disease, as well as the development of solid tumors, particularly ErbB-1 and ErbB-2 mediated.
- Insulin Receptor:
- Function: Crucial for regulating glucose metabolism, maintaining blood sugar levels, and overall metabolic balance.
- Platelet-derived Growth Factor Receptor (PDGFR):
- Function: Key role in cell growth and division, especially in tissue repair and wound healing.
- Vascular Endothelial Growth Factor Receptor (VEGFR):
- Structure: Extracellular segment comprises seven Ig-like domains, placing them within the immunoglobulin superfamily.
- Function: Critical for angiogenesis, the formation of blood vessels.
- Implications: Implicated in diseases such as cancer and vascular disorders.
- Fibroblast Growth Factor Receptor (FGFR):
- Diversity: Arises from four FGFR genes, generating more than 48 distinct isoforms through natural alternate splicing.
- Structure: Unified extracellular segment with three immunoglobulin-like domains (D1-D3), classifying them within the immunoglobulin superfamily.
- Function: Important for cell proliferation, tissue repair, and associated with developmental processes and tissue regeneration.
- Hepatocyte Growth Factor Receptor (c-Met):
- Function: Regulates cell movement (motility) and invasion, critical for tissue regeneration, particularly in the liver.
- Neurotrophic Tyrosine Kinase Receptors (TrkA, TrkB, TrkC):
- Function: Essential for the development and maintenance of the nervous system, playing a crucial role in neuronal survival, growth, and differentiation.
- Eph Receptors:
- Contributions: Contribute to cell migration, axon guidance, and synapse formation.
- Importance: Essential in the development and functioning of the nervous system.
- Anaplastic Lymphoma Kinase (ALK):
- Implications: Implicated in certain cancers, particularly in the nervous system.
- Role: Involved in abnormal cell growth and survival.
- ROS1 Receptor Tyrosine Kinase:
- Associations: Associated with various cancers, especially lung cancer.
- Function: Drives uncontrolled cell growth and contributes to tumor development.
General mechanism of action of Receptor tyrosine kinases (RTKs)
- Ligand Binding and Receptor Dimerization
- RTKs are activated through the binding of ligands, often forming dimeric ligand-receptor complexes.
- Ligand binding induces conformational changes that either stabilize receptor chains or expose binding domains, leading to dimerization.
- Trans-Phosphorylation and Activation
- Dimerization facilitates trans-phosphorylation of specific cytoplasmic tyrosines in the kinase domains of the receptors.
- In some cases, ligands induce conformational changes necessary for receptor dimerization.
- Constitutive Dimerization and Ligand-Induced Changes
- Some RTKs, like insulin receptors, exhibit constitutive dimerization, while others, like EGF receptors, require ligand-induced conformational changes for dimerization.
- Cis-Inhibition and Activation Loop Regulation
- In the absence of ligands, activation loops inhibit catalytic activity in a “closed” conformation (cis-inhibition).
- Ligand-induced dimerization leads to the rotation of lobes, exposing the activation loop for ATP binding and autophosphorylation.
- Trans-Phosphorylation and Stabilization
- Trans-phosphorylation of tyrosine residues in the activation loop stabilizes the “open” conformation, breaking the binding with protein substrates.
- Molecular “Brakes” and Kinase Activity Regulation
- Molecular domains in activation loops, juxtamembrane domains, or C-terminal domains act as “brakes” to limit phosphorylation levels.
- Ligand-induced conformational changes remove these molecular repressions through cis-phosphorylation.
- Tyrosine Phosphorylation and Intracellular Signal Transduction
- Phosphorylation of catalytic domains activates the kinase domain, while non-catalytic domains provide anchoring sites for cytoplasmic targets.
- Tyrosines on juxtamembrane and C-terminal domains allow the binding, activation, and phosphorylation of cytoplasmic proteins involved in intracellular signal transduction.
- Adapter and Anchoring Proteins
- SH2 or PTB domain-containing proteins recognize tyrosine-phosphorylated receptor chains and relay signals.
- Adapter proteins, like Grb2, link to the MAPK activation pathway, while anchoring proteins bind directly to receptor chains or adaptative proteins.
- Specific Binding and Multiple Tyrosine Residues
- Proteins such as Gab1 bind to specific phosphorylated tyrosine residues on receptor chains.
- Adaptive proteins, used by insulin and FGF receptors, assemble into phosphorylatable complexes.
Activation of RTKs by Dimerization
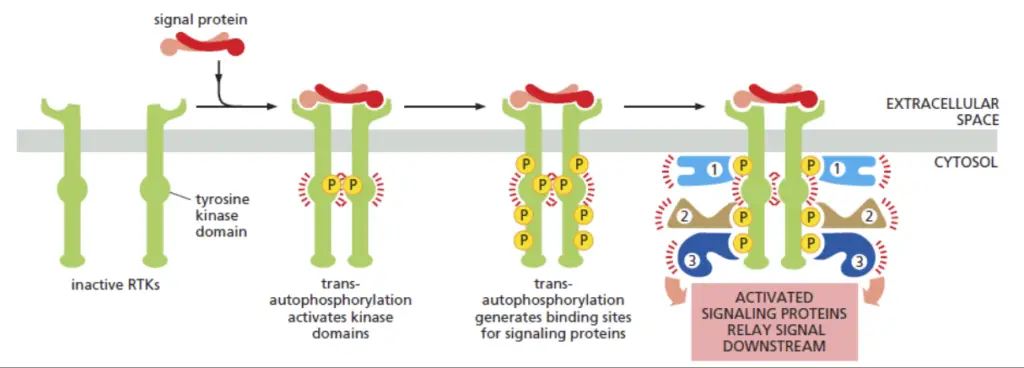
- Receptor Tyrosine Kinase (RTK) Structure: RTKs are transmembrane proteins, featuring extracellular and intracellular domains, with a transmembrane segment linking the two. The extracellular region is responsive to external signals, while the intracellular domain possesses kinase activity.
- Ligand Binding: Activation begins with the binding of specific signaling molecules, often growth factors or hormones, to the extracellular domains of RTKs. This binding is analogous to fitting keys (ligands) into locks (RTKs), triggering a conformational change in the receptor.
- Conformational Changes and Dimerization: Ligand binding induces conformational alterations in the RTKs, promoting their association in a dimeric configuration. This pairing occurs as a result of the spatial proximity facilitated by ligand-induced conformational changes.
- Transmembrane Connection: The transmembrane segment of RTKs ensures a secure connection between the dimerized receptors across the cell membrane. This structural feature anchors the RTKs in the cell membrane, facilitating their coordination upon dimerization.
- Initiation of Intracellular Signaling: Dimerization activates the intracellular domain of RTKs. This domain possesses kinase activity, allowing the RTK to undergo autophosphorylation. Autophosphorylation involves the addition of phosphate groups to specific tyrosine residues within the intracellular domain.
- Autophosphorylation: The autophosphorylation process serves as a molecular switch, enhancing the kinase activity of RTKs. The addition of phosphate groups not only activates the RTK itself but also creates docking sites for downstream signaling molecules.
- Propagation of Signaling Cascades: Phosphorylated RTKs initiate a cascade of intracellular signaling events. These events involve the phosphorylation of other intracellular proteins, creating a chain reaction that transduces the extracellular signal into the cell.
- Cellular Responses: The signaling cascades regulated by activated RTKs influence various cellular processes, including but not limited to cell growth, division, and survival. The specificity of these responses is determined by the nature of the ligands and the downstream effectors involved in the signaling pathway.
- Diverse Mechanisms of Kinase Activation: Dimerization induces kinase activity through diverse mechanisms. In some instances, such as the insulin receptor, it involves mutual phosphorylation of specific tyrosines within the kinase active sites. Conversely, in receptors like the epidermal growth factor (EGF) receptor, kinase activation is initiated by conformational changes resulting from interactions between the two kinase domains outside their active sites.
- Versatility of RTK Activation: The distinct mechanisms employed by RTKs during dimerization-induced activation highlight the versatility of these receptors in modulating cellular signaling pathways. A comprehensive understanding of these mechanisms contributes to unraveling the complexity of cellular responses to external stimuli.
RTKs and activated signalling pathways
Receptor Tyrosine Kinases (RTKs) serve as pivotal protein platforms initiating diverse cellular signaling pathways. These pathways, involving enzymatic effectors, adapter proteins, and downstream effectors, play crucial roles in regulating cellular processes. The primary downstream signaling pathways associated with RTK activation include MAPK, PI3K, Src, and others, with intricate mechanisms guiding signal transduction.
- MAPK Pathway:
- Initiation:
- Ligand-induced RTK activation leads to the recruitment of Grb2 and SOS adapter proteins.
- Grb2 binds to phosphorylated tyrosine residues, facilitating SOS activation.
- SOS activates Ras, a small G protein, by exchanging GDP for GTP, initiating downstream signaling.
- Propagation:
- Activated Ras recruits and phosphorylates Raf kinases, activating MEK1/2 and subsequently Erk1/2.
- Phosphorylated Erk1/2 translocates to the nucleus, regulating gene transcription for cell survival and growth.
- Targets:
- Transcription factors (STAT, Elk-1, CREB, H3 histone) activate early genes, influencing cell cycle progression.
- Initiation:
- Additional MAPK Pathways:
- p38 Pathway:
- Activated by MAP2K (MKK3 or MKK6), regulates cell proliferation, angiogenesis, and inflammation.
- JNK Pathway:
- Activated by MAP3Ks (TAK1, MEKK1, MLK), controls cell apoptosis and immune system development.
- ERK5 Pathway:
- Activated by WNK1, regulates cell proliferation and survival by controlling cyclin D1 expression.
- p38 Pathway:
- PI3K/Akt/mTOR Pathway:
- Activation:
- RTK activation recruits PI3K, initiating the phosphorylation of membrane lipids to form PIP3.
- Akt and PDK-1 bind to PIP3, leading to Akt phosphorylation and activation at the membrane.
- Functions:
- Akt inhibits pro-apoptotic proteins (BAD, p53) and induces anti-apoptotics (Bcl-2, Akt).
- Activated Akt promotes cell proliferation by regulating cyclins, inhibiting cell cycle repressors, and inducing pro-angiogenic genes.
- Activation:
- Src Pathway:
- Activation:
- Src family members (Src, Fyn, Yes) are activated by RTKs and associated with various kinases (Ras, PI3K, PLCγ).
- SFKs regulate intracellular signaling pathways, influencing DNA synthesis, cell survival, and motility.
- Mechanisms:
- SFKs bind phosphorylated residues, activating kinase activity and contributing to RTK activation.
- c-Src, within membrane complexes, phosphorylates RTKs, influencing signal transmission.
- Activation:
- Additional Signaling Pathways:
- PLCγ and JAK/STAT:
- RTKs activate PLCγ and JAK/STAT pathways.
- PLCγ is associated with processes like ubiquitination, glycosylation, and acetylation.
- JAK/STAT pathways involve binding of STAT transcription factors to phosphorylated tyrosine residues, leading to gene activation.
- PLCγ and JAK/STAT:
Pathway | Initiation | Propagation | Targets | Functions and Mechanisms |
---|---|---|---|---|
MAPK Pathway | Ligand-induced RTK activation | Activated Ras recruits and phosphorylates downstream kinases | Transcription factors (STAT, Elk-1, CREB, H3 histone) activate early genes | Ligand induces RTK activation, leading to Grb2 and SOS recruitment; Ras activation; Propagation through Raf, MEK, and Erk; Translocation of phosphorylated Erk to the nucleus; Transcription factor activation for cell survival and growth. |
p38 Pathway | Activated by MAP2K (MKK3 or MKK6) | Regulates cell proliferation, angiogenesis, and inflammation | Various genes involved in cell processes | Activation of p38 by MAP2K; Induction of gene transcription involved in cell proliferation, angiogenesis, and inflammation. |
JNK Pathway | Activated by MAP3Ks (TAK1, MEKK1, MLK) | Controls cell apoptosis and immune system development | Regulates apoptosis and immune system development | MAP3K activation leads to JNK activation; JNK controls cell apoptosis and immune system development. |
ERK5 Pathway | Activated by WNK1 | Regulates cell proliferation and survival | Transcription factors, pro-apoptotic proteins, etc. | WNK1 activation leads to MEKK2/3 activation, phosphorylation of MEK5, and activation of ERK5; ERK5 regulates cell proliferation, survival, and specific substrates. |
PI3K/Akt/mTOR Pathway | RTK activation recruits PI3K; Akt phosphorylation | Akt regulates cell survival, proliferation, and angiogenesis | Inhibits pro-apoptotic proteins, induces anti-apoptotics, regulates cell cycle | RTK-induced recruitment of PI3K and Akt activation; Akt-mediated inhibition of pro-apoptotic proteins and regulation of cell cycle and angiogenesis. |
Src Pathway | Activated by RTKs | Regulates DNA synthesis, cell survival, motility, etc. | Influences DNA synthesis, cell survival, and motility | Src family members activated by RTKs; Regulate DNA synthesis, cell survival, and motility; SFKs bind phosphorylated residues and contribute to RTK activation. |
PLCγ and JAK/STAT | RTKs activate PLCγ and JAK/STAT pathways | PLCγ associated with various cellular processes | JAK/STAT involves transcription factor activation and gene regulation | RTK-induced activation of PLCγ and JAK/STAT pathways; PLCγ associated with various cellular processes; JAK/STAT pathways involve transcription factor activation and gene regulation. |
RTKs in oncology
1. RTK Mutations and Carcinogenesis:
- RTKs, including MET, KIT, and FLT3, are implicated in approximately 30% of human cancers, displaying mutations or overexpression.
- Oncogenic mutations in KIT and FLT3, particularly in the juxtamembrane region, lead to constitutive receptor activation, directly contributing to carcinogenesis.
2. RTK Inhibitors and Bone Cancers:
- RTK Inhibitors Targeting the Bone Tumour Niche:
- Osteoblasts and osteoclasts express various RTKs, making them cellular targets in the cancer micro-environment.
- RTK inhibitors, such as lapatinib, erlotinib, and sunitinib, affect bone remodelling by modulating osteoblastic proliferation.
- Some kinase inhibitors, like GSK1838705A and masitinib, inhibit osteoblast viability, highlighting the complex effects of RTK inhibitors on bone cells.
- Therapeutic Benefits for Bone Sarcomas:
- Bone sarcomas, originating from mesenchymal stem cells, exhibit potential therapeutic targets in various RTKs.
- PDGFRα, Axl, EphB2, FGFR2, IGF-1R, and Ret are identified as potential targets for inhibitors like imatinib mesylate and pazotinib.
- Clinical investigations for RTK inhibitors (e.g., dasatinib, sunitinib, pazotinib) in bone sarcomas yield variable results, requiring tailored approaches based on RTK expression.
- Therapeutic Benefits for Bone Metastases:
- Bone metastases from breast or prostate carcinomas involve dysregulation of local bone remodelling, leading to investigations of RTK inhibitors.
- Clinical trials with inhibitors like imatinib mesylate, dasatinib, and sunitinib show varied results, often falling short of expected outcomes.
- Cabozantinib demonstrates promise in pre-clinical models but falls short in phase III clinical trials, emphasizing the complexity of targeting RTKs in bone metastases.
- Multiplicity of targets and the intricacies of the tumour microenvironment contribute to the challenges in achieving significant therapeutic outcomes.
3. Potential Therapeutic Targets in Bone Cancers:
- Newly Identified RTKs:
- Axl, EphA2, FGFR2, IGF-1R, and Ret emerge as newly identified RTKs with potential therapeutic benefits in bone sarcomas.
- Expression patterns of these RTKs vary across osteosarcoma and Ewing’s sarcoma, influencing clinical outcomes.
- Challenges and Considerations:
- Targeting RTKs in bone cancers demands consideration of expression/mutation/activation states, necessitating tailored clinical investigations.
- Clinical trials with various RTK inhibitors reveal the need for nuanced approaches, considering the tumour microenvironment’s impact on drug efficacy.
Regulation of Receptor tyrosine kinases (RTKs)
The intricacies of the receptor tyrosine kinase (RTK) pathway are meticulously governed by an array of positive and negative feedback loops to maintain cellular homeostasis and prevent aberrations such as cancer and fibrosis.
1. Protein Tyrosine Phosphatases (PTPs):
- Protein Tyrosine Phosphatases (PTPs) form a crucial group of enzymes with a catalytic domain possessing phosphotyrosine-specific phosphohydrolase activity.
- These enzymes play a dual role in the regulation of RTK activity, both positively and negatively.
- PTPs act by dephosphorylating activated tyrosine residues on RTKs, effectively terminating the signaling cascade.
- PTP1B, a prominent member, participates in cell cycle regulation and cytokine receptor signaling, demonstrating its role in dephosphorylating receptors like the epidermal growth factor receptor and the insulin receptor.
- Some PTPs, like Cd45, serve as cell surface receptors contributing positively to cell signaling and proliferation by facilitating the dephosphorylation of specific phosphotyrosines that inhibit the Src pathway.
2. Herstatin:
- Herstatin, an autoinhibitor of the ErbB family, assumes a pivotal role in the RTK regulation by binding to RTKs and impeding receptor dimerization and tyrosine phosphorylation.
- Experimental data, such as CHO cells transfected with herstatin, reveal reduced receptor oligomerization, clonal growth, and receptor tyrosine phosphorylation in response to EGF.
3. Receptor Endocytosis:
- Activated RTKs employ endocytosis as a mechanism for downregulating the receptor and subsequently dampening the signaling cascade.
- This process involves the engulfing of the RTK through clathrin-mediated endocytosis, culminating in intracellular degradation.
Three major pathways can transduce a signal from activated RTK
1. Ras-MAP Kinase Pathway
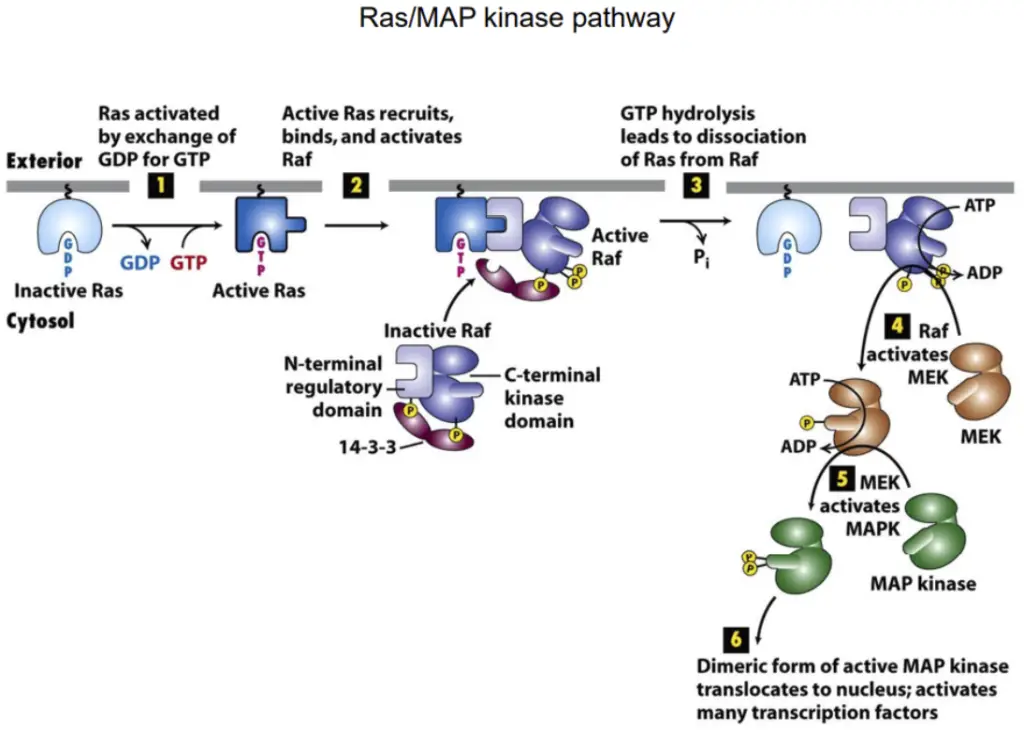
Comprises various families of monomeric GTPases transmitting signals from cell-surface receptors. Serves as signaling hubs by interacting with intracellular proteins.
- Ras Proteins in Humans:
- Three major Ras proteins: H-, K-, and N-Ras.
- Anchored to the cell membrane, relay signals for cell functions, including proliferation.
- Role of Ras in Cancer:
- Inhibition of Ras function impedes cell responses to activated receptors.
- Hyperactive Ras mutants in 30% of human tumors contribute to uncontrolled cell proliferation.
- Ras as a Molecular Switch:
- Functions by cycling between active (GTP bound) and inactive (GDP bound) states.
- Regulated by Ras guanine nucleotide exchange factors (Ras-GEFs) and Ras GTPase-activating proteins (Ras-GAPs).
- Activation of Ras by RTKs:
- RTKs activate Ras through Ras-GEFs, with Son-of-sevenless (Sos) identified as a Ras-GEF in genetic studies.
- Adaptor protein Grb2 links RTKs to Sos, facilitating Ras activation.
- Downstream Signaling:
- Activated Ras triggers downstream signaling proteins, essential for cell proliferation and differentiation.
- Ras-MAP Kinase Pathway Mechanism:
- The pathway involves a conserved sequence of Ras → Raf → MEK → MAP kinase (ERK).
- Ras activates Raf, which, in turn, activates MEK, leading to the phosphorylation of MAP kinase.
- Despite variations in biological outcomes, the Ras → Raf → MEK → MAP kinase sequence is conserved across organisms.
- The pathway regulates cellular responses, including cell proliferation and differentiation.
2. IP3/DAG Pathway
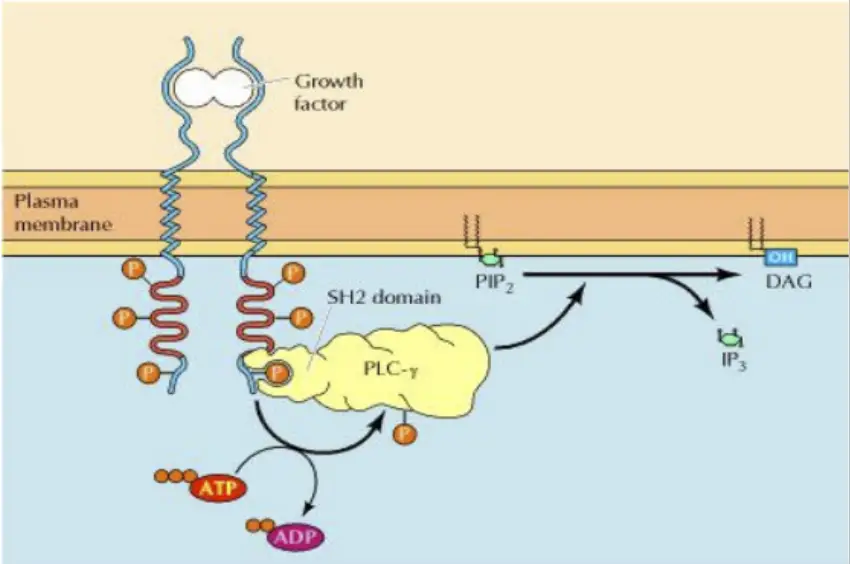
- Involves a series of events responding to extracellular signals.
- Begins with the binding of a signaling molecule to a cell membrane receptor, activating phospholipase C γ (PLC γ).
- PLC acts on the membrane lipid phosphatidylinositol 4,5-bisphosphate (PIP2), generating IP3 and DAG.
- IP3 triggers the release of intracellular calcium ions (Ca2+), influencing various cellular responses.
- DAG activates protein kinase C (PKC), modifying target protein activity through phosphorylation.
- Orchestrates cellular responses by regulating intracellular calcium levels and activating PKC.
- Crucial in neuronal signaling, impacting neurotransmitter release and synaptic plasticity.
3. PI-3 Kinase Pathway/AKT Pathway
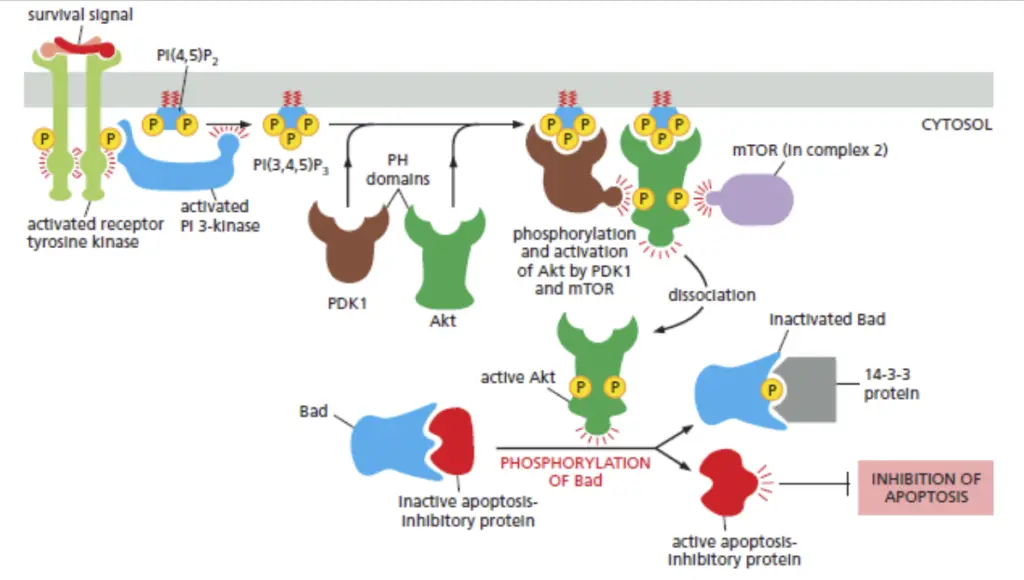
- Also known as the AKT pathway, regulating cell survival, growth, and metabolism.
- Initiated by the activation of Phosphoinositide 3-kinase (PI3K) in response to external signals.
- PI3K generates PIP3, serving as a docking site for AKT, recruiting it to the cell membrane.
- Activated AKT phosphorylates downstream proteins, including mTOR, influencing cell growth and protein synthesis.
- Tight regulation by negative regulators like PTEN and modulation through feedback loops ensure balanced responses to extracellular signals.
- Diverse isoforms of PI3K and AKT contribute to pathway diversity, each with specific roles in different tissues and cellular contexts.
These three major pathways in RTK signaling play crucial roles in governing cellular responses, ensuring intricate control over processes such as proliferation, differentiation, and survival. The orchestration of these pathways reflects the complexity and adaptability of cellular signaling networks.
Important facts about Signalling by RTK pathway
- RTK Activation and Phosphorylation:
- Activation of the kinase domains in an RTK dimer initiates phosphorylation in cytosolic regions outside the kinase domain.
- Phosphorylation creates high-affinity docking sites for intracellular signaling proteins.
- Signaling proteins selectively bind to specific phosphorylated sites on the activated receptors, facilitated by phosphotyrosine-binding domains.
- Formation of Intracellular Signaling Complex:
- Receptor phosphorylation serves as a molecular switch, triggering the assembly of an intracellular signaling complex.
- The complex transduces signals along multiple pathways, directing cellular responses to diverse destinations.
- Different RTKs activate distinct responses by engaging different combinations of signaling proteins within the complex.
- Role of Docking Proteins:
- Some RTKs utilize docking proteins, such as IRS1, to enhance the signaling complex.
- IRS1 associates with phosphorylated tyrosines on the activated receptor, creating additional docking sites through its own phosphorylation.
- Variety of Intracellular Signaling Proteins:
- Numerous signaling proteins, including enzymes like PLCγ, Src, and PI 3-kinase, bind to phosphotyrosines on RTKs.
- These proteins contribute to signal relay, culminating in diverse cellular responses.
- Phosphotyrosine-Binding Domains:
- Signaling proteins commonly feature conserved phosphotyrosine-binding domains, such as SH2 or PTB domains.
- These domains enable specific binding to activated RTKs and other phosphorylated proteins, facilitating the formation of signaling complexes.
- Feedback Mechanisms:
- Proteins with SH2 domains may engage in negative feedback mechanisms, down-regulating RTK signaling.
- Example: c-Cbl catalyzes the ubiquitylation of receptors, promoting their endocytosis and subsequent degradation.
- Endocytosis and Signaling:
- Ligand-induced endocytosis of RTKs does not uniformly decrease signaling.
- RTKs can persistently signal from endosomes or intracellular compartments, enabling long-distance communication, as observed in nerve growth factor (NGF) signaling.
- Adaptor Proteins:
- Some signaling proteins function as adaptors, characterized by SH2 and SH3 domains.
- Adaptor proteins facilitate interactions between tyrosine-phosphorylated proteins and those lacking SH2 domains, coupling them to downstream signaling proteins like Ras.
Functions of Receptor tyrosine kinases (RTKs)
- Cell Growth and Proliferation:
- RTKs are central regulators of cell growth and proliferation by initiating signaling cascades that lead to the activation of pathways promoting cell cycle progression.
- Cell Differentiation:
- RTK signaling is crucial for directing cellular differentiation, guiding undifferentiated cells into specialized cell types with distinct functions and characteristics.
- Cell Survival and Apoptosis:
- RTKs contribute to cell survival by activating pathways that inhibit apoptosis (programmed cell death). Conversely, the downregulation of RTK signaling can trigger apoptotic processes.
- Metabolism Regulation:
- RTKs are involved in regulating cellular metabolism by influencing processes such as glucose uptake and utilization. For instance, insulin receptor, an RTK, plays a critical role in glucose metabolism.
- Tissue Repair and Wound Healing:
- RTKs participate in tissue repair and wound healing processes by promoting cell migration, proliferation, and differentiation at the site of injury.
- Immune System Function:
- RTKs are involved in immune system responses, influencing the activation and function of immune cells. They play roles in immune cell differentiation, migration, and cytokine production.
- Neuronal Development and Function:
- RTKs are crucial for the development and functioning of the nervous system. They regulate neuronal survival, growth, and differentiation, contributing to processes like synaptic plasticity and learning.
- Angiogenesis:
- RTKs are key regulators of angiogenesis, the formation of new blood vessels. Vascular Endothelial Growth Factor Receptor (VEGFR), a type of RTK, plays a central role in this process.
- Hormone Signaling:
- RTKs mediate signaling in response to various hormones, influencing endocrine functions and hormonal regulation of physiological processes.
- Embryonic Development:
- RTKs are critical during embryonic development, guiding cellular processes such as organogenesis and tissue patterning.
- Response to Environmental Stimuli:
- RTKs act as sensors, responding to extracellular signals such as growth factors, hormones, and cytokines, translating these signals into intracellular responses.
- Regulation of Gene Expression:
- RTK signaling pathways often influence gene expression by activating transcription factors, thereby modulating the synthesis of proteins involved in cellular functions.
Applications/Uses of Receptor tyrosine kinases (RTKs)
- Biomedical Research:
- Cell Signaling Studies: RTKs are crucial in understanding cell signaling pathways. Their study provides insights into the molecular mechanisms governing cell growth, differentiation, and survival.
- Disease Mechanisms: Investigating aberrant RTK signaling is integral to understanding the underlying molecular mechanisms of various diseases, including cancer, neurodegenerative disorders, and metabolic diseases.
- Drug Discovery and Development:
- Target Identification: RTKs are prime targets for drug discovery efforts. Identifying specific RTKs involved in disease processes allows for the development of targeted therapies.
- Therapeutic Development: Drugs targeting RTKs, known as kinase inhibitors, have been developed for diseases like cancer. Examples include tyrosine kinase inhibitors used in the treatment of certain types of leukemia and solid tumors.
- Cancer Diagnostics and Prognostics:
- Biomarker Identification: Aberrant expression or mutation of RTKs is often associated with cancer. Detection of specific RTK alterations serves as diagnostic and prognostic biomarkers.
- Targeted Therapies: Drugs targeting specific RTKs, such as EGFR inhibitors or HER2-targeted therapies, are employed in cancer treatment based on the molecular profile of tumors.
- Neuroscience Research:
- Nervous System Development: Studying RTKs is essential for understanding the development of the nervous system, including neuronal survival, axon guidance, and synapse formation.
- Neurological Disorders: Aberrant RTK signaling is implicated in neurological disorders. Research on RTKs provides insights into potential therapeutic targets for conditions like Alzheimer’s and Parkinson’s diseases.
- Cardiovascular Research:
- Angiogenesis Studies: RTKs, particularly VEGFR, are critical for angiogenesis. Investigating these receptors is essential for understanding blood vessel formation and cardiovascular health.
- Vascular Disorders: Dysregulation of RTKs is associated with vascular disorders. Targeting RTKs is explored for potential therapeutic interventions in conditions like atherosclerosis.
- Regenerative Medicine:
- Tissue Regeneration: Understanding RTK signaling pathways contributes to knowledge about tissue regeneration. Manipulating RTK activity may be explored for enhancing tissue repair and regeneration.
- Stem Cell Research: RTKs play roles in the regulation of stem cell differentiation and maintenance. Research in this area has implications for regenerative medicine and tissue engineering.
- Endocrinology and Metabolism:
- Insulin Signaling: RTKs, such as the insulin receptor, are central to insulin signaling and glucose metabolism. Research in this area informs understanding of metabolic disorders like diabetes.
- Hormonal Regulation: RTKs mediate signaling in response to various hormones, influencing endocrine functions and hormonal regulation of physiological processes.
- Clinical Diagnostics:
- Mutation Analysis: Genetic mutations in RTKs are assessed for diagnostic purposes. Mutation analysis aids in determining disease susceptibility and prognosis.